Engineering Microscale Disease Models for Heart and Skeletal Muscle
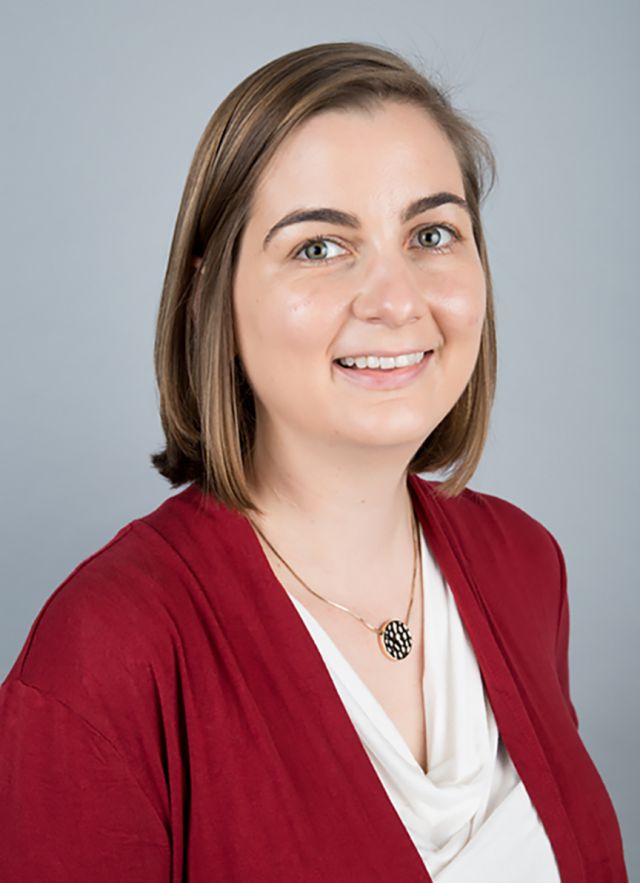
Speaker
Megan McCain, Ph.D.
Chonette Early Career Chair Assistant
Professor of Biomedical Engineering and
Stem Cell Biology and Regenerative Medicine
University of Southern California
Abstract
The most common forms of heart disease are acquired from changes in the biomechanical loading of the heart or adverse cardiac tissue remodeling triggered by an injury, such as a myocardial infarction. In contrast, most skeletal myopathies, such as amyotrophic lateral sclerosis, are caused by genetic mutations. Regardless of their etiology, most diseases that afflict the heart and/or skeletal muscle involve muscle cells as well as other cells in the tissue that regulate muscle cell function. These diseases often lead to heart failure or loss of mobility, respectively, by a variety of mechanisms that are incompletely understood due largely to the limitations of existing model systems. Thus, we need new experimental models that allow users to: (1) precisely tune biomechanical forces, tissue architecture, and cell-cell interactions; (2) visually interrogate dynamic processes in living muscle cells and tissues; (3) quantify relevant physiological outputs, including excitation-contraction coupling; and (4) measure the effects of patient-relevant genetic mutations. In this talk, I will describe how we engineer microscale models of healthy and diseased cardiac and skeletal muscle tissues tailored to these design parameters and implement them to achieve new insights into heart and skeletal muscle physiology and pathophysiology.
Bio
Megan L. McCain, PhD, is the Chonette Early Career Chair and an Assistant Professor in the Departments of Biomedical Engineering at USC Viterbi School of Engineering and Stem Cell Biology and Regenerative Medicine at Keck School of Medicine of USC. Her research group, the Laboratory for Living Systems Engineering, integrates tunable biomaterials, microfabrication techniques, and multiple cell types to engineer microscale mimics of human tissues that are easy to interrogate, known as “Microphysiological Systems” or “Organs on Chips”. They implement these constructs primarily to model and understand the progression of cardiac and skeletal muscle diseases. Megan is a recipient of a Scientist Development Grant from the American Heart Association and an Innovation Award from the Eli and Edythe Broad Foundation. She has also been recognized as a 2014 Top Innovator Under 35 by MIT Technology Review and a 2018 Young Innovator of Cellular and Molecular Bioengineering by the Biomedical Engineering Society. She received her PhD and postdoctoral training in Engineering Sciences from Harvard University under Prof. Kevin Kit Parker.